What Nobel Prize winners moves
Four outstanding German scientists take a look into the future.
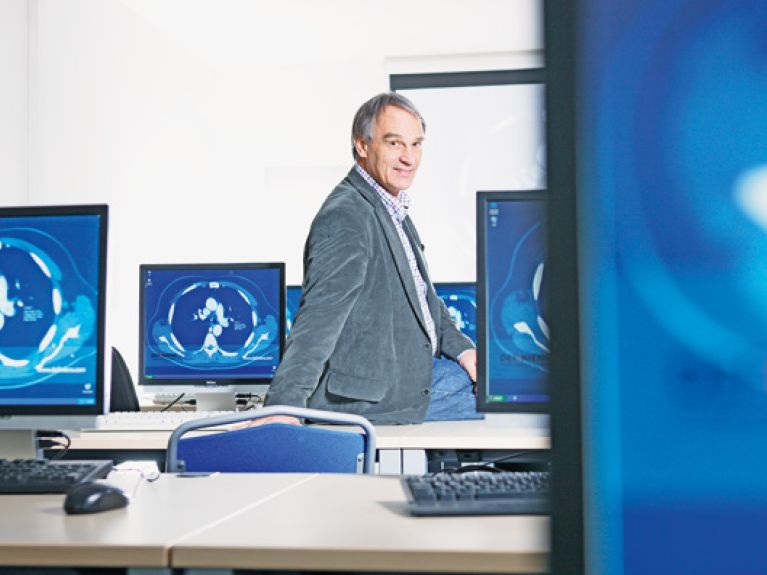
Gerd Binnig: the best of both worlds
There are piles of boxes stacked in his office: Definiens, Gerd Binnig’s company, is moving to another location in Munich. That rather typifies a researcher who has remained on the move. Binnig received the 1986 Physics Nobel Prize for the groundbreaking invention of the scanning tunnelling microscope, and today Definiens uses the latest software for image analysis.
“Today we are already successfully using our method of software-based image analysis in biological and medical research. Our software is based less upon abstract algorithms than on the way in which humans observe and understand images. A few years ago, for example, it was considered inconceivable that a computer could analyze a tissue section. That’s possible using our method, which demonstrates the enormous potential of image analysis. In the future we must more strongly bring together the best of both worlds: the speed and precision of machines and the strength of humans to recognize links through their ability to understand images. The software of the future will be essentially influenced by knowledge of individual disciplines. In research under certain circumstances a medical scientist can programme better than a computer scientist because he or she is closer to the actual challenge. Software will continue to distance itself from technical requirements and to a certain extent become more human.”
The scanning tunnelling microscope developed by Binnig and Swiss scientist Heinrich Rohrer enables insights into the world of nanostructures. The future opportunities today seem enormous: “Nanotechnology is opening a door to a new world. In my opinion, we are in the midst of a second genesis. In the first genesis, molecular structures and eventually diverse life forms developed from atoms. Today we have the chance of developing enormously complex structures out of the minutest nanocomponents. A long road lies ahead of us. Yet nanomedicine in particular could play a great role in the future. Take cancer, for example: we will have to penetrate even deeper into nanostructures in the future just to be able to understand the disease – and new therapeutic approaches could also be effective at that level.”
Mastering and harnessing complexity is the challenge that Gerd Binnig also associates with the vision of a powerful nanocomputer: “We must understand the structures of the nanoworld even better in order to be able to build one. Powerful nanocomputers could one day help in such different and complex challenges as global economic crises and the treatment of tumours. Computers of this kind could distribute innumerable specialized tasks among different computer elements. You have to imagine them as a network of specialized computers working together cooperatively on the smallest possible space. It would be ideal if we could succeed in doing this in a three-dimensional way with an exceptionally high degree of networking – following the model of the brain. It would then be possible to develop a computer that would eclipse anything achieved before.”
Hartmut Michel: the sun’s potential
We meet him in a city of the future: Science City Riedberg is the name of a new district of Frankfurt am Main where construction workers are busily building one structure after another. Hartmut Michel, who received the Chemistry Nobel Prize in 1988 for his part in deciphering photosynthesis, is engaged in research here into very special compounds: membrane proteins.
“The structural determination of membrane proteins holds great potential for the development of new medicines. We do not know the signal molecules of roughly 350 of the approximately 800 membrane proteins in the human body that pass signals into the cell interior as so-called G protein-coupled receptors. If we succeed in determining the functions of these receptors, they can be used as the basis for developing new drugs and therapies. The possibilities are already being demonstrated by receptor blockers designed to stop the growth of specific forms of breast cancer. There is also speculation that declining communication between the cell nucleus and the mitochondria could be a cause of aging. This forms the basis of the hope that in the future we will be able to combat degenerative diseases and perhaps even slow down the aging process by improving the coordination between cell nucleus and mitochondria.”
However, fathoming the finest cellular processes demands methodical expertise and ingenious high technology. Hartmut Michel outlines the prospects: “I expect a great deal from the sequencing of tumour genomes. Comparisons with healthy tissue can make it possible to discover key mutations and then block activated proteins in the tumour in a targeted way. We face two major challenges, however, in the structural determination of membrane proteins. We have so far only been able to obtain adequate quantities of very few membrane proteins. In addition, these proteins have to be stable enough to allow us to carry out the complicated crystallization process, which could possibly be obviated through the use of free-electron lasers with extremely intense X-rays. One of these extraordinarily powerful lasers is already working in Stanford in the USA, and one will soon be deployed in Hamburg. Bombarding individual molecules with such intense beams of X-rays makes it possible to record diffraction patterns and this could allow structural determination without previous crystallization, thereby greatly accelerating the process.”
The live-giving process of photosynthesis has already been deciphered – also thanks to Hartmut Michel, who can imagine innovations in this field too: “The efficiency of photosynthesis is very low: less than 1% of the energy of the sunlight is stored as biomass. The capacity of photosynthesis is already exhausted at 20% of the full energy of the sunlight, which means that 80% can no longer be used. If scientists were able to successfully reduce the size of light-harvesting complexes, this modification could significantly boost agricultural yields. The question of storage is crucial for the production of renewable energy. I see great opportunities here for new kinds of batteries – for example, on the basis of tin-lithium-sulphur – that offer many times the storage capacity of conventional lithium batteries.”
Theodor W. Hänsch: the uncertainty of our view of the world
You can see how much he delights in discoveries. His face lights up at the thought of a teetering model of the physical universe. He takes equal pleasure in outlining the potential of precision spectroscopy. Theodor Hänsch was honoured with the 2005 Physics Nobel Prize for the fundamental refinement of this measuring technology, primarily through the invention of the frequency comb.
“We are developing increasingly exact measuring tools. On the basis of the frequency comb technique we are using the frequencies of light as a high-precision pulse generator in optical clocks. And these measuring instruments are steadily becoming more practical. Ten years ago you still required an entire building to be able to measure the frequency of light. Today a desktop apparatus will do the job. Perhaps in the future we will be able to use light clocks of this kind that are the size of a matchbox. The fact that measuring devices are becoming ever smaller and ever more robust opens up new application opportunities – for example, in space travel, where the frequency comb technique will probably soon be used in satellite navigation systems. Basically, we hope that the continuing development of the most precise clocks will create greater benefit in situations where they are already important today. That does not only mean that satellite navigation can become even more precise, but it is also conceivable that more exact clocks would make it possible to improve the synchronization of mobile telephones, which would make mobile telecommunications even more powerful. Frequency combs are also used in astronomical research, because they can measure spectral lines – in other words, the light from faraway planets. The European Southern Observatory in La Silla, Chile, plans to use a frequency comb from 2013 in order to detect possible Earth-like planets.”
Yet Theodor Hänsch believes space is not the only thing that might hold surprises. Our entire model of the physical world could change: “Perhaps we are in a similar phase today as physicists were at the end of the 19th century. At that time many thought that all the important discoveries had been made and they fully accepted the state of knowledge then, for example, in the fields of mechanics and electrodynamics. Yet there were already first portents of revolutionary changes. Then came Einstein’s Theory of Relativity, which fundamentally transformed our understanding of time and space. Subsequently, quantum physics gave rise to completely new rules. Today, too, there are indications that our model of the world could change fundamentally. Phenomena like dark energy and dark matter do not fit in seamlessly with this model. Theoreticians are speculating on how we can reconcile gravitation and quantum physics. On the other hand, I am trying, as an experimental physicist, to detect discrepancies in the standard model with the aid of increasingly precise measuring methods. For example, these measuring methods have also been able to prove that the natural constants we know today are actually not so constant after all.”
Erwin Neher: the key to knowledge
Erwin Neher only needs to draw a few lines in chalk to explain the secrets of the human cell to his guest. “Simple, but ingenious” are words that are frequently used to describe the patch-clamp technique developed by Neher and Bert Sakmann. Their innovation revealed the function of ion channels in cells and brought the two researchers the 1991 Nobel Prize for Physiology or Medicine.
“My department at the Max Planck Institute for Biophysical Chemistry in Göttingen is currently engaged in research on neurotransmitters, the messenger substances that are effective through the synapses of our nerve cells. Here we are concentrating on a synapse in the auditory pathway, which is where the key lies to valuable insights into the processing of acoustic signals. We still do not have an adequate understanding of the hearing process. Perhaps our investigations will contribute to an explanation of phenomena like directional hearing. Since we are working on a synapse that is typical for the nervous system as a whole, we hope to gain a better general understanding – also of processes like learning and memory. Incidentally, most incurable illnesses are degenerative diseases of the nervous system, such as Alzheimer’s. They are incurable because we don’t understand the nervous system. Therefore our work could also make a promising contribution here.”
Using the patch-clamp technique, Neher and Sakmann succeeded in isolating a minute ion channel for the first time and deciphering cellular communication. Neher envisages various opportunities in the search for ever deeper insights: “I see special potential in the work of my Göttingen-based colleague Stefan Hell, who has now reached the nanometre scale with his enhancement of the optical microscope. We created the basis for measuring cells’ smallest signals with the patch-clamp technique 30 years ago. Yet even here there is still potential for further development. Nanion, a Munich-based biotech company, has developed a chip-based automatic patch-clamp procedure that makes it possible to test countless active agents in the shortest possible time. This will significantly advance the development of new medical drugs.”
Erwin Neher sees additional future potential in the study of artificially mutated ion channels: “Many hereditary diseases can be traced back to mutated ion channels. We can ‘re-create’ these mutations in an animal model, study their changed function and, as a result, draw important conclusions about the development of hereditary diseases, which could lead to new therapeutic approaches. It has already been possible to develop a therapy against infant diabetes in this way – and perhaps we can conquer other diseases using similar methods.”
Reports: Johannes Göbel